Boilogy
At school biology was taught to us as the science of life. At ARI Steamline, BOILogy is the science of boilers!! Here we will study everything from boiler evolution to boiler efficiency calculations.
A boiler is a closed vessel made of steel designed to heat water and generate steam above atmospheric pressure. The boiler consists of a compartment where the fuel is burned and a compartment where water can be evaporated into steam.
We heat the water, it evaporates to steam and the resultant expansion, pressurizes the system. Steam is thus, forced out of the boiler by its own pressure. The steam thus generated can be used for the development of motive power in a reciprocatng steam engine or steam turbine, or for use in process industries.
Boiler evolution.
In the 17th century, the events of the Industrial Revolution, primarily in England, promoted the rapid development of the steam engine by such inventors as Thomas Newcomen and James Watt.
Watt is credited with being the first inventor to separate the steam engine, and the boiler, into two separate units in the latter part of the 18th Century. In these early times, the primary use of the boiler was to generate steam for steam driven engines. As steam driven engines replaced the horse, as a means of motive power, it followed that steam driven engines were rated in ‘Horsepower’.
Boiler design progressed from what was essentially a kettle to a relatively large-diameter flue pipe submerged in water – thus the first fire-tube boiler. As power and pressure requirements increased, boilers became larger and the single-flue pipe became a larger number of smaller diameter flue tubes combined with an external, or internal, furnace for the combustion of the fuel.
Competition between fire-tube boiler manufacturers eventually forced improvements in boiler design and fuel burning equipment. This, together with a broad shift towards liquid and gaseous fuel utilization, resulted in cleaner and more reliable combustion and improved heat transfer within the boiler.
The basic evolution in boiler designs were:
- Plain Cylinder Boiler
- Cornish Boiler
- Lancashire Boiler
- Upright Fire Tube Boilers
- Scotch Boiler
The Plain Cylinder Boiler 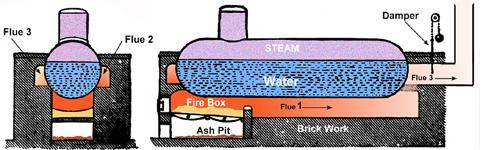
Fig: A Plain Cylinder Boiler
The first advancement in boiler design came with the invention of the Plain Cylinder Boiler.
It was a simple design and easily constructed.As its name implies, the Plain Cylinder Boiler is a long metal cylinder with conical (round) ends set horizontally in a brick work. The cylinder was half filled with water and a fire ignited in furnace at one end.
The fire and hot gasses are first channeled from the furnace or fire box along the bottom of the cylinder to the opposite end of the boiler. This channel is called a "flue" and is made of brick on three sides. The other side of the flue is the metal wall of the boiler. The flames and hot gasses touch the bare metal and heat the water inside the boiler.
When the hot gasses get to the end of the first flue, they are channeled back along one side of the cylinder to the front of the boiler. From there they are again channeled back along the other side of the cylinder to the chimney. This would give a boiler 40 feet long 120 feet of heating surface.
The speed with which the fuel burned was controlled by a damper near the chimney. Raising or lowering the damper controlled the draught or amount of air being drawn into the furnace. More air made the fuel burn faster and hotter making more steam. Less air saved fuel and produced less steam.
Although this boiler design was far more efficient than previous boilers, and had been used for more than one-hundred years, it had two major flaws.
1. The first was dirt. Water contained dirt and as water evaporated, this dirt collected in the bottom of the cylinder and acted like an insulator preventing the heat from reaching the water. Therefore, more fuel was burnt and more cleaning was required.
2. The second flaw was more dangerous. As the hot gasses traveled along the 120 foot long flue, they cooled a little. The metal of the cylinder was heated unevenly on either of the three sides causing stress leading to frequent explosions.
The Cornish Boiler
The invention of a workable steam boiler and engine made mass transportation possible. However hazardous it might have been. Economics was the driving force behind a new design - the Cornish Boiler.
Until that time, designers had always placed the furnace beneath the water cylinder. Then some genius had the idea of putting the fire where it would do the most good, in with the water. Not actually "IN" the water but literally inside the cylinder containing the water.
The Cornish Boiler made several design changes
1. First the 3 feet dia furnace was placed inside a metal tube in the boiler. This greatly increased the amount of heat transferred to the water.
2. The order in which the gasses moved was changed. After leaving flue #1 (the main furnace) the hot gasses were divided at the back into two streams - flue #2 which brings the gas back to the front.
3. At the front of the boiler the hot gasses were directed downward into flue #3 and traveled back beneath the boiler to the chimney. This helped reduce the amount of mud that accumulated in the bottom of the boiler and that increased the boilers efficiency even more.
4. The cylinder now had flat ends because of above design changes.
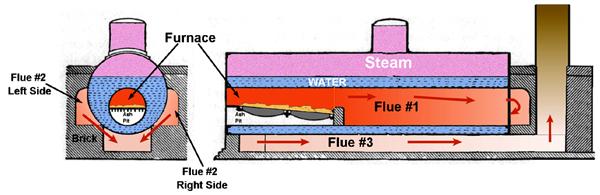
Fig: A Cornish Boiler
Unfortunately, the internal tube with its furnace and fierce heat was constantly changing length. It would bulge and then contract as the temp dropped even slightly leading to stress. The results were the same - an explosion!
The Lancashire Boiler The need for smaller more powerful, to say nothing of safer, steam boilers led to the Lancashire Boiler design. It had many advantages:
1. First, each boiler had two completely separate furnaces sitting side by side. And each furnace had a separate flue system. This idea was outstanding. Why?
Everything that burns contains some water. This water must be evaporated for fuel to burn efficiently. Doing this, the furnace cools somewhat and that in turn lowers the amount of air being drawn into the furnace. The less air drawn in the less heat created in the furnace. This slight cooling placed a heavy strain on the ends of the boiler. It also slowed the heating of the water inside the boiler reducing the amount of steam available.
With the Lancashire Boiler each furnace is stoked at a different time. This means that one furnace is always producing maximum heat and that heat creates a powerful draught in both furnaces speeding up the ignition process. Something like blowing on a campfire. It heats things up real fast.
It also means that more air is drawn into the system which allows combustion (burning) of the smoke created by the "low" burning furnace. This combustion takes place in flue #2 thereby increasing the amount of heating of the sides of the cylinder.
2. Like the boilers we have already seen, the Lancashire Boiler has three flues. But the Lancashire Boiler is designed with two separate flue systems. Gasses from the right side furnace remain on the right side of the boiler while hot gasses from the left furnace remain on the left side. They do not combine until they reach the base of the chimney connection. This system provided a very powerful, even and constant draught in both furnaces.
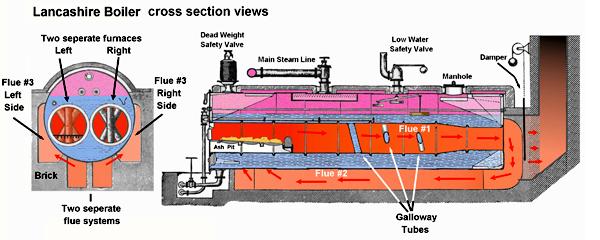
Fig: A Lancashire Boiler
3. Another major improvement in heat transfer and fuel efficiency was the addition of "Galloway Tubes". Hollow metal tubes which traverse (connect both sides of ) the main flue #1. Water in the boiler flowed through these tubes which are subject to heating by the hottest fire and gasses which pass around them.
4. The increased efficiency of Lancashire style boilers also allowed them to be smaller. Commonly only seven feet in diameter (side to side) and twenty-seven feet long. A great saving of both space and weight.
5. There were extensive number of internal braces designed to keep the cylinder from rupturing. The braces, and stays kept the ends from bulging and added much to the overall strength of the boiler.
6. These boilers were the first to feature the "low water safety valve" shown in the illustration. Should the water level drop below the top of the internal flues, the intense heat of the furnace would quickly burn through the metal. A float rides up and down with the water level in the boiler. As the water dropped below a predetermined level, the valve would gradually open and release steam pressure. The noise this valve made when it opened would also get the attention of the engineer very, very, quickly.
7. A second "Pop Off Safety Valve" was also installed at the front of the boiler. These valves operated on steam pressure alone. When the pressure in the boiler exceeded a specified amount, these valves suddenly "popped" wide open and stayed wide open until they were reset or replaced.
They can still be seen in some old paper plants. The concept of riveting came from these boilers (in the past, the welding quantity was not good enough for higher pressures)
The Upright Fire Tube Boilers
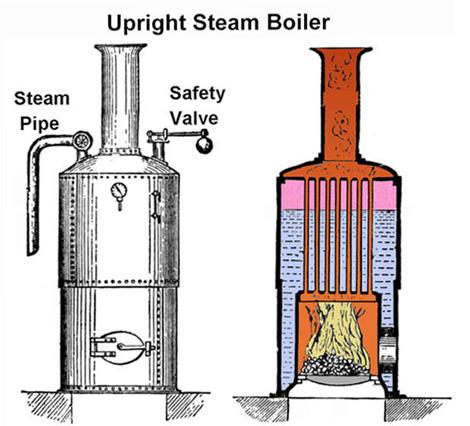
We take a side track and explain something about the vertical (upright) boiler.
- The furnace is located inside the water tank. It has a number of brass tubes which extend through the boiler to the chimney carrying the hot gases allowing an extremely high rate of heat transfer to the water. In other words, the fire passed through the tubes, hence the name, Fire Tube Boiler.
- The water tank or boiler is a vertical tank not a horizontal cylinder.
- Their compactness and the speed with which they developed a working steam pressure was of major importance.
- The relatively low pressures developed by this style of boiler made it perfect for many jobs - from heating a home to powering small steamboats.
Disadvantages:
- The extremely hot gasses made only one pass through the boiler so this design is not as efficient as those that route the heat back and forth. Much heat was wasted going straight up the chimney.
The Scotch Boiler (2-pass) Engineers and designers of steam boilers had long understood the relationship between the amount of heat generated in a furnace and the ability of water to absorb that heat. Yet, unlike the Lancashire boiler, the Scotch boiler does
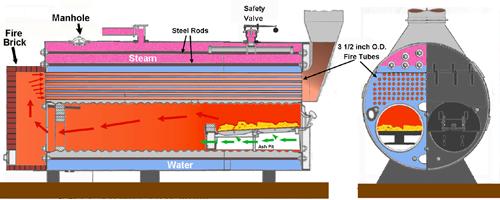
Fig: A 2-pass Scotch Boiler
- The water tank is made from corrugated plates and does not utilize Galloway tubes. Because of the larger water surface exposed to the heat more heat is transferred to the water.
- The end plates are reinforced by heavy through bolts. This combination of through bolts and corrugated plates provided an extremely strong boiler.
- The Scotch Boiler has “fire tubes” arranged above the furnaces, but below the water surface.
- At the back end of the boiler the hot gasses entered a chamber, or Dry Back which allowed the end plate to be heated and also directed the gasses into the fire tubes. From there the hot gasses moved forward through the numerous tubes to the chimney.
- The Scotch Boiler was quite versatile. Designs were built to deliver anywhere from 6 to 300 BHP (boiler horse power).
Disadvantages: - Water circulation within the boiler was poor. Cooler water was settling at the bottom of the boiler, acting like an insulator and decreasing the efficiency of the boiler.
- It also allowed mud and scales to be deposited on the outside of the main flues.
- The metal tubes could not transfer heat effectively to the water. Eventually the insulation effect would allow the metal to heat to a point where it would bend.
Boiler classification
There are two approaches in boiler design: fire tube and water tube. The goal in all cases is to maximize the heat transfer between the water and the hot gases heating it.
Fire-tube boilers
The name firetube is very descriptive. The fire, or hot flue gases from the burner, is channeled through tubes that are surrounded by the fluid to be heated. The tubes in a fire-tube boiler are made of carbon steel. The body of the boiler is the pressure vessel and contains the fluid. In most cases this fluid is water that will be circulated for heating purposes or converted to steam for process use.
Every set of tubes that the flue gas travels through, before it makes a turn, is considered a "pass". So a three-pass boiler will have three sets of tubes with the stack outlet located on the rear of the boiler. A 4-pass will have four sets and the stack outlet at the front. A fire-tube boiler was more common in the 1800s.
It consists of a tank of water perforated with pipes. The hot gases from a coal or wood fire run through the pipes to heat the water in the tank, as shown here:
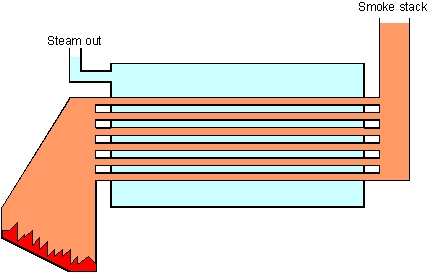
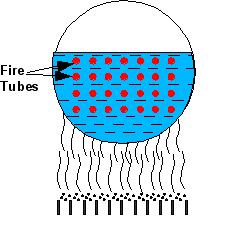
Advantages: - Relatively inexpensive
- Easy to clean
- Compact in size
- Available in sizes from 600,000 btu/hr to 50,000,000 btu/hr
- Easy to replace tubes
- Well suited for space heating and industrial process applications
Disadvantages: - Not suitable for high pressure applications 250 psig and above
- Limitation for high capacity steam generation
- In a fire-tube boiler, the entire tank is under pressure, so if the tank bursts it creates a major explosion.
For example, steam locomotives have fire-tube boilers, where the fire is inside the tube and the water on the outside. These usually take the form of a set of straight tubes passing through the boiler through which hot combustion gases flows.
Water-tube boilers A Watertube design is the exact opposite of a fire tube. Here the water flows through a rack of narrow tubes that are encased in a furnace in which the burner fires into. The tubes frequently have a large number of bends and sometimes fins to maximize the surface area. These tubes are connected to a steam drum and a mud drum. The water is heated and steam is produced in the upper drum.
These boilers are more common today. This type of boiler is generally preferred in high pressure applications since the high pressure water/steam is contained within narrow pipes which can contain the pressure with a thinner wall.
Large steam users are also better suited for the Water tube design. The industrial watertube boiler typically produces steam or hot water primarily for industrial process applications, and is used less frequently for heating applications.
Advantages: - Available in sizes that are far greater than the firetube design. Up to several million pounds per hour of steam.
- Able to handle higher pressures up to 5,000 psig
- Recover faster than their firetube cousin
- Have the ability to reach very high temperatures
Disadvantages: - High initial capital cost
- Cleaning is more difficult due to the design
- No commonality between tubes
- Physical size may be an issue
The following simplified diagram shows you a typical layout for a water-tube boiler:
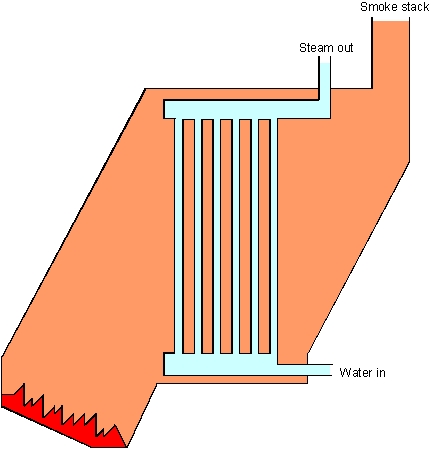
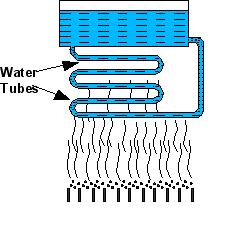
In a real boiler, things would be much more complicated because the goal of the boiler is to extract every possible bit of heat from the burning fuel to improve efficiency.
The older fire-tube boiler design—in which the water surrounds the heat source and the gases from combustion pass through tubes through the water space—is a much weaker structure and is rarely used for pressures above 350 psi (2.4 MPa). A significant advantage of the water tube boiler is that there is less chance of a catastrophic failure: There is not a large volume of water in the boiler nor are there large mechanical elements subject to failure.
Here are some other ways to classify a boiler.
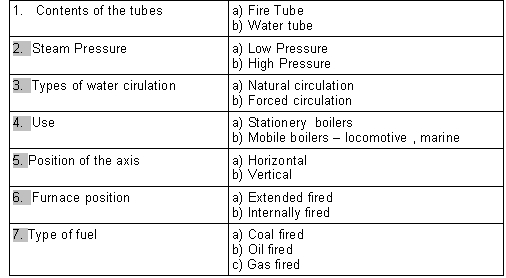
Table: Classification of boilers
Types of fuels.
Sources of heat for the boiler can be the combustion of fuels such as wood, bagasse, coal, oil or natural gas. Electric boilers use resistance or immersion type heating elements. Nuclear fission is also used as a heat source for generating steam. Waste-heat boilers, or HRSGs use the heat rejected from other processes such as gas turbines.
Boilers are now specifically designed to utilize a wide range of standard and alternative fuels.
Solids: Coals like Bitumen and Anthracite
Liquids: Oils like FO/LDO/ LSHS / HSD
Gas: Natural gas/ LPG
Alternative fuels: Agricultural waste like bagasse, husk, shells / wood / shavings
FO (Furnace Oil - this fuel is a heavy, unrefined fuel and is less than half the cost of diesel)
LDO (Light Diesel Oil)
LSHS (Low Sulphur Heavy Stock means it is less polluting)
HSD (High Speed Diesel)
Natural gas This is piped to the plant.
With the development of fluidised bed combustion, the solid fuel boiler's efficiencies have gone up considerably. Therefore, a lot of plants are now looking at solid fuels.
Waste Heat There are some application which are exothermic in nature - like, DG sets, reactors. Their waste water is generally cooled before being released into rivers or sewage pipes. Instead, we now use this waste heat to pre-heat water for steam.
Fuel Selection Fuel is selected based on the following parameters:
- GCV(Gross Calorific Value)
- Availability
- Cost
- Manageability
- Emissions
- Byproducts
- Boiler Design
- Boiler Efficiency (η) Fuels
GCV (Gross Calorific Value). This tells us how much heat we get by burning the fuel.
Availability and cost. It is important that the fuel is available easily and at a reasonable cost.
Manageability. Fuels like coal or bagasse, are more difficult to transport, store and feed.
Emmissions from boilers have become a cause for concern in Mumbai. The eastern side of Mumbai is switching to natural gas-fired boilers as there are residences coming up in Mulund.
Coal has a lot of by-products, especially coal dust. These are undesirable by-products especially for food or pharma plants.
Boiler design. Obviously the choice of design determines what type of boiler is used. It is the fuel which is decided first, as the
cost of fuel used per year is always more than the boiler cost
Efficiency(η) based on GCV Lancashire – (50-60)%
2 P – 70%
3 P W B (oil) – 85%
FBC - (70 -75)%
Chain grate - (60-70)%
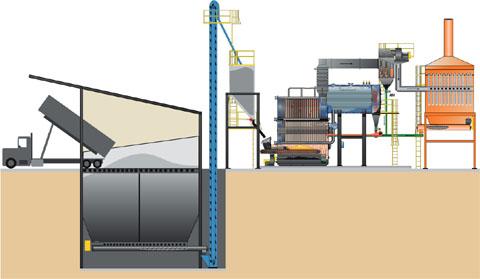
Fig. Chain grate for a coal Boiler
Modern Boilers.
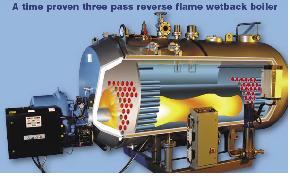
Fig: A 3-pass reverse flame wetback boiler
The modern boilers are far superior to these old giants. They are smaller, cheaper, with high efficiencies and the heat release rates are higher, so smaller surface areas are needed for heat transfer.
Reverse flame The combustion chamber is shaped like a cylinder but narrow at the far end. This way, when the burner fires down the centre (1st pass), the flame doubles back on itself within the combustion chamber (2nd pass) to come out at the front of the boiler. The flue gases now enter the fire tubes surrounding combustion chamber, and go to the rear (3rd pass) of the boiler and the chimney. It has an economizer to pre-heat the boiler water where flue gases are used to pre-heat the water.
Water-tube boilers The water-tube boiler was patented in 1867 by American inventors George Herman Babcock and Stephen Wilcox. In the water-tube boiler, water flowed through tubes heated externally by combustion gases, and steam was collected above in a drum. Water tube boilers are very huge and their water holding capacity is enormous. The water-tube boiler became the standard for all large boilers as they allowed for higher pressures than earlier boilers, higher than 30 bar. Example, Babcock & Wilcox boiler manufactured at Thermax Boilers Ltd., Pune.
It is a horizontal, externally fired, stationary, high pressure, water tube boiler with a super heater as shown below.
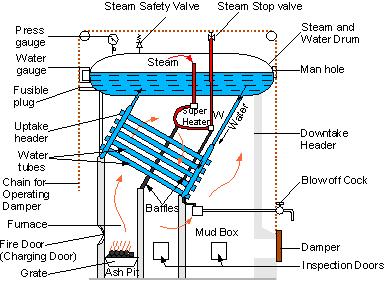
The coal is fed from hopper on to the grate where it is burnt. The flue gases are deflected by the fire brick baffles so that they pass across the left side of the tubes in a beneficial path transferring heat to water in the tubes and to the steam in the super heater and finally they escape into the atmosphere through the chimney. The drought is regulated by a damper placed at the back chamber.
The position of water tubes near the furnace is heated to a higher temper than the rest. Owing to higher temperature, the density of water decreases and hence the water rises through the uptake header and short tube to the drum. The water at the back end, which is at a lesser temperature now travels down through the long tube and the downtake header. Thus, a continuous circulation of water called as natural circulation is established between the water tubes and the drum. The steam produced gets collected above the water in the drum.Here, saturated steam is drawn off the top of the drum.
Since water droplets can severely damage turbine blades, dry steam from the steamdrum is again heated to generate superheated steam at 730°F (390°C) or higher in order to ensure that there is no water entrained in the steam. Cool water at the bottom of the steam drum returns to the feedwater drum via large-bore 'downcomer tubes', where it helps pre-heat the feedwater supply. To increase the economy of the boiler, the exhaust gasses are also used to pre-heat the air blown into the furnace and warm the feedwater supply. Such water-tube boilers in thermal power station are also called steam generating units.
NIBR Boilers
In many small plants, the use of Non IBR Coil type boilers has proliferated, mainly due to the following perceived benefits:
- Lower initial cost
- Equivalent efficiency as IBR boilers
- No IBR approved boiler operators are required
- No “hassle” of annual boiler inspection by IBR
- No IBR piping required
Maintenance
A coil boiler requires very stringent water quality to be maintained. The coil requires frequent maintenance/replacements in view of water quality normally not being maintained. Manufacturers of coil-type non-IBR boilers do a roaring business in spare coils.
Safety
In absence of any proper guidelines/inspecting authority/control (such as IBR inspection), quality of fabrication itself is suspect. Shell (IBR) boilers have much better controls. IBR boilers have controls in their manufacturing process which automatically bring with them a higher level of safety.
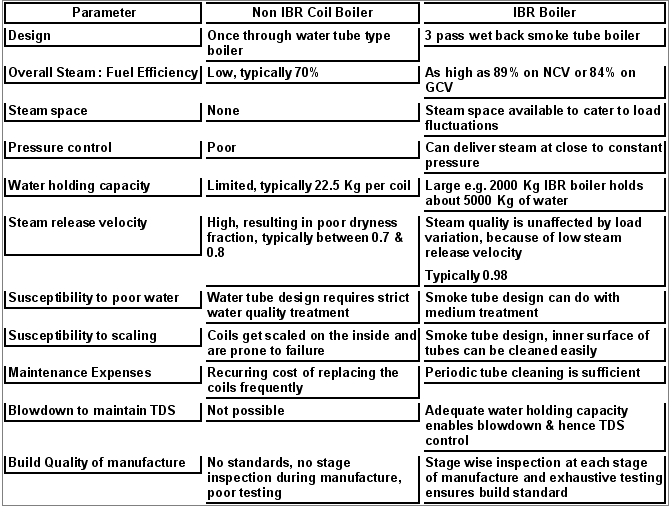
3PWB package We will study this in detail, as this is the most common boiler we will come across.
The 3PWB packaged boiler
The 3PWB packaged boiler is the most common type of boiler in most installations. The main components of the 3PWB boiler are shown as under.
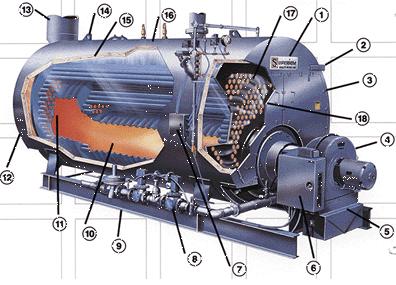
Pic: A Three-pass Wetback Boiler
- Manufacturers name plate This has the MCR - Maximum Continuous Rating – of the boiler mentioned on it, besides the F&A rating.
- Boiler shell. This stores water for heating. It is cylindrical in shape with both ends closed.
- Front and rear doors are closed using studs with lugs and brass nuts for ease of opening and closing. These doors allow for total access to the return tubes.
- Burner. An equipment which burns the fuel. Burners mix air with fuel to provide oxygen in the combustion process. Burners are specifically matched with the furnace diameter and lenght for complete/efficient combustion. Burners utilise gas, light/ heavy oil or pulverized (finely ground) coal in combination with air or pressure atomization of the oil.
- Air louver, linkage and setting is visuable for ease of monitoring, adjustment and cleaning.
- Control panel. This is used to control the boiler and is either boiler mounted or on a separate skid
- Control circuit junction box with terminal strips to permit checking individual control operations.
- Fuel oil heating and filtering systems.
- Structural steel skid type base supports the boiler and protects burner. This is bolted to the foundation.
- Furnace. Space in a boiler where a burner burns liquid or gas fuel. (A fire grate burns solid fuel). The hot flue gases travel from furnace to the chimney. A large diameter furnace will provide for complete combustion and maximum heat transfer.
- Wetback turnaround eliminates the need for refractory lining, bafles, gaskets and provides additional primary heating surface for incrased efficiency.
- Split/hinged rear doors provide access to the rear tube sheet and third pass tubes.
- Round rear smoke outlet.
- Heavy steel lifting eyes for ease of handling.
- Mineral fiber insulation reduces heat loss through the jacket and provides jacket support.
- Hard enamel paint finishto the galvanized coated steel jacket.
- 3 Pass design for optimum efficiency and economical operation.
- Front smokebox and doors lined with ceramic fiber blanket.
Chimney. This is a system for venting hot gases and smoke from a boiler to the outside atmosphere. They are typically almost vertical to ensure the hot gases flow smoothly, drawing air into the combustion through convection. The space inside a chimney is called a flue. Chimneys are tall to disperse pollutants in the exhaust over a greater area reducing the concentration of toxins to a safe level and to increase the draw.
On and around a boiler
The heart of any modern steam system is the boiler house. Here we need to achieve
- maximum Safety via Boiler mountings
- high Efficiency and economical running via Boiler auxillaries
- excellent Controls on pressure and temp (see Boiler controls)
Boiler mountings - for maximum safety
All the following are mounted on the boiler shell and are a must for every boiler. All of them are provided for the safe working of boiler.
- Feed pumps
- Feed check valve
- Main steam stop valve(MSSV) or Crown valve
- Mobrey - water level indicator
- Safety Valve
- Gauge glass
- Fusible plug

Fig: Boiler with various mountings
Feed check valve
The boiler feed check valve is designed specifically for use on boiler feedwater systems. The valve is opened by the boiler feedwater pressure and is closed by its spring as soon as the flow ceases, preventing reverse flow. The strong spring supports the head of water in an elevated feedtank when there is no pressure in the boiler, preventing the boiler from flooding. It is a normally a stainless steel disc check valve to ensure tight shut-off against boiler pressure, even under poor water conditions.
Main Steam Stop valve (MSSV) or Crown valve
This valve isolates the boiler and its steam pressure from the process or plant. Always open this slowly to prevent a drastic increase in downstream pressure. Other dangers of opening the Main steam stop valve quickly is priming, where slugs of water may enter the distribution system or waterhammer, where collected condensate slugs in the distribution pipe picks up sudden velocity. It should not be used as a pressure reducing valve to throttle steam flow, but should be in fully open or closed mode.
Mobrey / Water level indicator
This is a level switch. This is an extremely important safety mechanism which maintains water level above the fire-tubes at all times. If the water level falls below the tubes, heat cannot be dissipated to water. The temperatures within the fire tube build up and the tubes can melt.
Most accidents happen because of a fall in water level in the boiler!!
In fact in most cases there is a second low water level alarm interlocked to the burner. This shuts off the burner as soon as the water level falls below a specified limit.
When we want to shut down a boiler, we cut off the burner and then start the feed pumps to cool down the water in the boiler.
Safety ValveA safety relief valve is one of the most critical safety devices on any boiler. It is the boiler’s last measure of protection against overpressure. It must be adequately sized and of the correct pressure rating for the boiler.
But getting a safe installation is only the beginning. The safety relief valve also must be inspected and tested regularly. Mud and scale from the boiler can interfere with the operation of the relief valve. Plugged discharge lines can prevent proper operation or allow discharged water and steam to come in contact with equipment or operating personnel.
Lifting the test lever while the boiler is operating will confirm its proper operation. At no time should technicians test the valve by increasing the pressure of the boiler to a level higher than the safety-valve setting. They should exercise caution when testing relief valves, as steam or hot water will be discharged through the valve at the operating pressure of the boiler. Valves should be tested every time a boiler is started and at the interval recommended by the manufacturer.
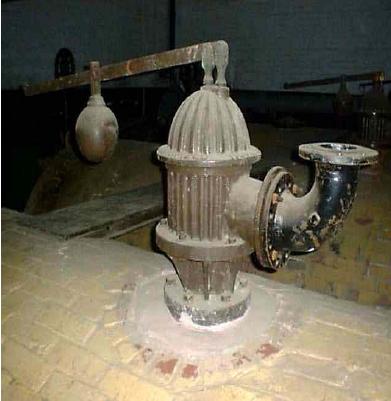

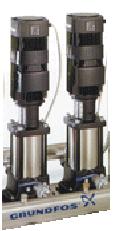
A deadweight safety valve on top of a boiler. The valve lifting pressure is set by the movement of the weight to the left of the arm. The further the weight from the valve, the higher the pressure in the boiler required to release the excess steam. Gauge glass The glass tube, or pair of flat glass plates, fitted to a water-gauge to provide a visual indication of the water-level in a boiler or a tank.
A simple clear glass tube is mounted between two shut off valves which are connected to the rear of the boiler by pipes. When both valves are opened, the tube fills with water and steam. The surface of the water in the tube is precisely at the same level as the water in the boiler. You know in an instant how much water the boiler is holding.
Feed pumpsA pump that supplies water to a boiler. In most modern-age boilers, these are vertical, multi-stage pumps to provide the high pressures required.
Fusible plug A hollowed threaded plug having the hollowed portion filled with a low melting point material used, for example, in the crown of a boiler firebox. If the water level falls below them the metal in the plug melts and steam is dumped into the firebox to prevent serious overheating of the plates.
Boiler auxillaries - for high efficiency and economical running
These are used to improve the efficiency of the boiler. An economiser, super heater and air pre-heater are the main accessories. These are not a must for any boiler but are highly desirable.
- Economisers
- Super-heaters
- Air pre-heaters
Economisers An economiser is a device fitted to a boiler which saves energy by using the exhaust gases from the boiler to pre-heat the cold water used the fill it (the feed water).
Flue gases from large boilers are typically 250 - 350°C. Stack Economizers recover some of this heat for pre-heating water. The water is most often used for boiler make-up water or some other need that coincides with boiler operation. Stack Economizers should be considered as an efficiency measure when large amounts of make-up water are used (ie: not all condensate is returned to the boiler or large amounts of live steam are used in the process so there is no condensate to return.)
It consists of an array of vertical cast iron tubes connected to a tank of water above and below, between which the boiler's exhaust gases are passed. This is the reverse arrangement to that of fire tubes in a boiler itself; there the hot gases pass through tubes immersed in water, whereas in an economiser the water passes through tubes surrounded by hot gases. For good efficiencies, the tubes must be free of deposits of soot.
They are often referred to as feedwater heaters and heat the condensate from turbines before it is pumped to the boilers.
The savings potential is based on the existing stack temperature, the volume of make-up water needed, and the hours of operation. Economizers are available in a wide range of sizes, from small coil-like units to very large waste heat recovery boilers.
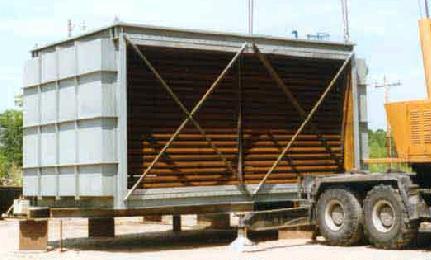
Stack Economizers should be considered as an efficiency measure when large amounts of make-up water are used (ie: not all condensate is returned to the boiler or large amounts of live steam is used in the process so there is no condensate to return) or there is a simultaneous need for large volumes of hot water.
Super-heaters
A superheater is a device that heats the steam generated by the boiler again, increasing its thermal energy and decreasing the likelihood that it will condense inside the engine.
Superheat refers to the process of increasing the temperature of steam above saturation temperatures to produce a very "dry" steam with absolutely no water vapor. This feature is most common in very large power plant boilers of watertube construction. Power plants use superheated steam to run the turbine blades. Turbine blades are very vulnerable to water damage. Super heated steam being absolutely dry, is much more suited for this expensive equipment, increasing its life and reducing replacement costs.
Air pre-heaters
An air preheater is designed to heat air before combustion in a boiler. The purpose of the air preheater is to recover the heat from the flue gas from the boiler to improve boiler efficiency by burning warm air which increases combustion efficiency, and reducing useful heat lost from the flue. As a consequence, the gases are also sent to the chimney or stack at a lower temperature, allowing simplified design of the ducting and stack. It also allows control over the temperature of gases leaving the stack (to meet emissions regulations, for example).
It uses waste heat to pre-heat air for combustion in boilers. Better combustion can be achieved as the fuel can be atomized better after pre-heating of air.
Efficiency and losses
A modern steam boiler will generally operate at an efficiency of between 80 and 85%. Some distribution losses will be incurred in the pipework between the boiler and the process plant equipment, but for a system insulated to current standards, this loss should not exceed 5% of the total heat content of the steam. Heat can be recovered from blowdown, flash steam can be used for low pressure applications, and condensate is returned to the boiler feedtank. If an economiser is fitted in the boiler flue, the overall efficiency of a centralised steam plant will be around 87%.
There are two methods to calculate boiler efficiency.
Direct Method 
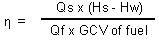
where,
Qs = Quantity of steam (in kg/hr)
Hs = Heat contained in steam at operating pressure (in kcal/kg)
Hw = Heat of feedwater (in kcal/kg)
Qf = Quantity of fuel (in kg/hr)
GCV (Gross Calorific Value) =Energy contained in fuel in kcal/kg
Indirect Method We calculate boiler efficiency by the Indirect Method using the BS 845 / ASME PTC 4.1 standards.
Boiler η =100 – losses,
=100 – (L1 + L2 + L3 + -------------+ Ln)
Here L1, L2, etc are the various losses.
Boiler losses
Let us take a look at these losses from and around the boiler.
- GCV vs NCV
- Stack loss
- Radiation loss
GCV LossThere is a difference in the gross calorific valve (GCV) - theoretical ; and Nett Calorific Valve (NCV) - actual of fuel. This is because of two reasons.
- The fuel contain moisture and when burnt, first the heat,makes the moisture in fuel evaporate and then the heat is used to heat water.
- The fuel contains hydrogen, which also has to be burnt
In furnace oil (FO) the difference (loss) between GCV and NCV is 6.25% (typically).
Stack LossThe flue gases lost to the atmosphere from chimney is called the stack loss. (The chimney is called stack as earlier bricks were stacked to make a chimney).
It is a function of the ΔT between the temperature of the flue gases (Tg) and the ambient temperature (Ta).
Stack loss is dependent on the difference(Tg – Ta). Stack losses can be as high as 10%, or be optimised to about 5%.
Radiation Loss Even though the boiler surface is insulated with 3''- 4'' of insulation material, there is still a radiation loss taking place. In a lot of older boilers, in fact, the insulations may be old & not working. Typically, based on boiler loading, radiation loss is 1-4%.
Boiler controls
We must have an excellent control on pressure and temperature as well as other parameters in the boiler.
- Sequence control
- Feedwater level control
- Pressure (firing) control
- Trim control
- Blowdown control
Sequence control Every boiler has a sequence of events to be executed before start-up and after shut down. To illustrate, a simple start-up sequence for an oil-fired boiler is shown.
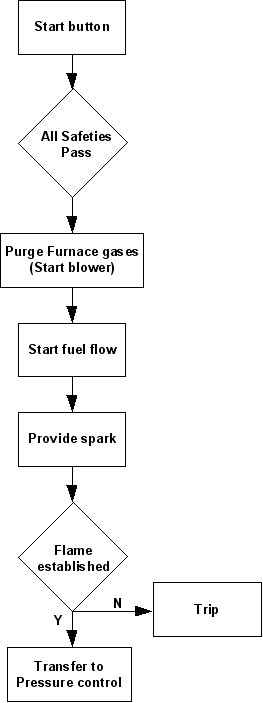
Feedwater level control The purpose of feedwater control system is to maintain the corect water level in the boiler under all load conditions.
Feedwater level control must be able to regulate water level under very dynamic conditions when the heat rate changes in the boiler cause the boiler level to shrink and swell, due to steam bubble volume changes in response to iring rate changes.
It also has to respond to momentary changes in steam demand replacing steam that has left the boiler with feedwater.
If the feedwater control system fails we are looking at serious problems. A high water level can cause serious damage to the distribution system and to m/c such as turbines. A low water level can expose the boiler tubes allowing the high flame temperatures to weaken and melt the boiler steel causing a catastrophic high energy release of steam from inside the boiler.
A level control system usually employs probes which sense the level of water in the boiler. At a certain level, a controller will send a signal to the feedpump which will operate to restore the water level, switching off when a predetermined level is reached. The probe will incorporate levels at which the pump is switched on and off, and at which low or high level alarms are activated. Alternative systems use floats.
Pressure (firing) control
Energy is supplied to the boiler via a combustion process and the combustion control system regulates the firing rate by controlling the amount of air and fuel delivered to the burners.
Combustion control systems are regulated to maintain the desired steam pressure and they must be able to respond to the many fluctuating conditions of the burner, fuel and air control sub-systems in a co-ordinated way to maintain the steam pressure set point in spite of varying process demands.
Trim control or, Air-fuel ratio control Air is comprised of approximately 21% oxygen and 79% nitrogen. When air is delivered for combustion, the nitrogen absorbs heat and is carried up the stack, resulting in energy losses. If there is excess air, the result is unused oxygen as well as even more nitrogen to absorb heat that is carried up the stack.
Boiler efficiency can be improved by incorporating an excess air trim loop into the boiler controls. It is easy to detect and monitor excess air, as oxygen not used for combustion is heated and discharged with the exhaust gases. A stack gas oxygen analyzer can be installed to continuously monitor excess air and adjust the boiler fuel-to-air ratio for optimum efficiency.
Performance/Costs: An often-stated rule of thumb is that boiler efficiency can be increased by 1% for each 15% reduction in excess air or 20°C reduction in stack gas temperature. An annual fuel savings of 5% is often obtained with tighter excess air control.
You can periodically “tune” your boiler and manually optimize fuel-to-air ratios after measuring the oxygen in the flue gas with an inexpensive test kit. More expensive hand held computer-based analyzers display percent oxygen, stack gas temperature, and boiler combustion efficiency. An automatic oxygen trim control system minimizes operating costs through ensuring that the proper fuel-to-air mixture is maintained at all boiler loads.
Chemistry Excess Air in Chemistry.
Blowdown control
Rather than control blowdown manually,
Continuous blowdown, sometimes called surface or skimmer blowdown, is more effective in controlling the concentration in boiler water. Where continuous blowdown systems are used, bottom blowdown is used for removal of precipitated impurities, especially those that tend to settle in the lower parts of the boiler.
Blowdown is also controlled to eliminate energy wastage - which is possible if more water than is necessary is dumped from the operating boiler - quite possible in case of manual blowdown.
Heat exchangers can be used with continuous blowdown, to recover energy from this expelled boiler water.
F&A rating
The boiler is rated to work at a certain pressure and at that pressure it can generate a defined quantity of steam. This can also be written as the F & A rating of the boiler.
This is used as a measure of the boiler ability to produce steam. It gives us the amount of steam (in kgs) that a boiler will produce if supplied with water at 100ºC. eg. A 2 ton boiler gives us 2000 kgs of steam per hour. It is written as 2 TPH F&A 100.
This means that when the water inside the boiler is at 100ºC and the steam take-off is also at 100ºC, the boiler will gives us 2 tons/hour of steam.
F&A Rating X 540 = Actual Rating X ( hg – hfFW) where,
hg = enthalpy of steam at generation pressure
hfFW = Feed water enthalpy
Example 6.1. Take a 10 TPH boiler, @ 10.54 barg, 75°C

= 9152 kg/hr nett
So, a 10TPH boiler will never give you 10,000 kg/hr of steam.
This disparity is because the feed is not at 100 deg C. Water at 100ºC has 100 kcal /kg latent heat. The F&A rating assumes an ideal condition:
+ 540 kcal/kg
100 kcal/k ----------------------------> 640 kcal/kg
(water at 100°C) (+heat) (steam's latent heat)
But actually, the feedwater is at ambient temperature. So we need more heat to first raise the temperature to saturation (100°C). This is what gives rise to the difference in boiler output.
+ 610 kcal/kg
30 kcal/k ----------------------------> 640 kcal/kg
(water at 30°C) (+heat) (steam's latent heat)
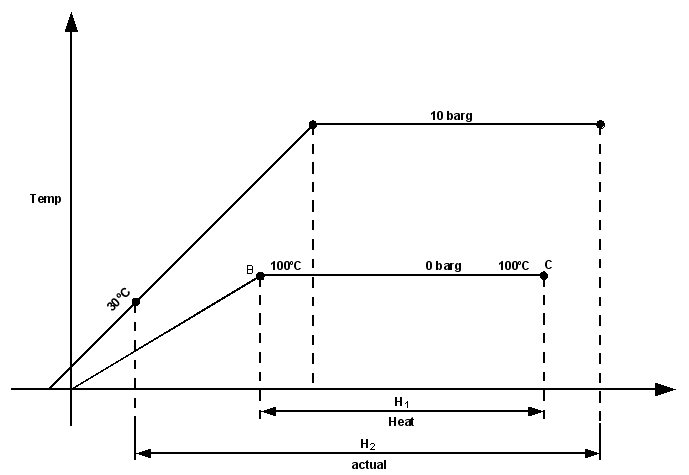
FAQs
Question 1: What is the steam space / water holding of a boiler ?
Any boiler must be designed so that it can carry the largest volume of water possible keeping adequate steam space.
If there is a larger body of water at close to saturation, any sudden loading of the boiler (switching on a new process in the plant) will not impact the pressure. It can give off huge steam loads intermittently. On the other hand, if there is a smaller quantity of water in the boiler, sudden load increase could drop the steam pressure.
A minimum steam space of 25-30% of diameter of boiler is needed. This is because, the closer you bring water to the top of the boiler, the greater the chances of carry over inside a boiler as there is a lot of turbulence at the separation surface. Bubbles of steam are rising up and bursting, so water droplets might enter the system along with steam. That means if the steam space is too small, dryness fraction decreases.
For a similar reason, a larger furnace is often fitted to a boiler. It can withstand thermal shocks which occur when there is a sudden rise in steam demand.
Question 2: Shouldn't we do a 4th pass to get higher efficiency ?
In fire tube boilers, the temperatures of the various passes are :
1st Pass - 700 to 1100
2nd Pass - 400 to 700
3rd Pass - 200 to 300
Normally, we generate steam at 10 bar (187ºC) so in a 4th pass the water will heat the fuel. Instead of a 4th pass, we can use the fuel gases to pre-heat the fuel (economizer).
Question 3: Why should stack temperature rise? Is it because excess air blows out unburnt fuel and gases? No. in fact, excess air will only decrease the temperature even if it is blowing out unburnt fuel and gases.
Stack temperatures rise mostly when the tube in the boiler cannot provie efficient heat transfer because of soot on tubes, or oil inside the tubes. The water cannot therefore, absorb all the heat being generated in the firetubes and the end result is a lot of heat lost via the stack . Hence the rise in stack temperatures. Inadequate heat transfer area within the boilers will also result in elevated stack temperature levels.
Water salts and scale deposits on tube from outside also impede heat transfer. Boilers using water from DM plants need less cleaning as compared to boilers using well water.
Fouling of the heat transfer area also occurs because of unclean fuel.
Question 4: Why should dampers for excess air levels be watched ?
As the seasons change, the air density also changes. In summer, for example, the air is less dense as compared to winters. This is because when it gets hot, the volume increases. So, we may need to ramp up excess air levels during the summers as compared to winters.
Also, the damper is a mechanical movable part. So it may lose its calibration. A pocket O2 analyzers will help us set the damper to a correct level. (On line O2 analyzers are also available but they are expensive).